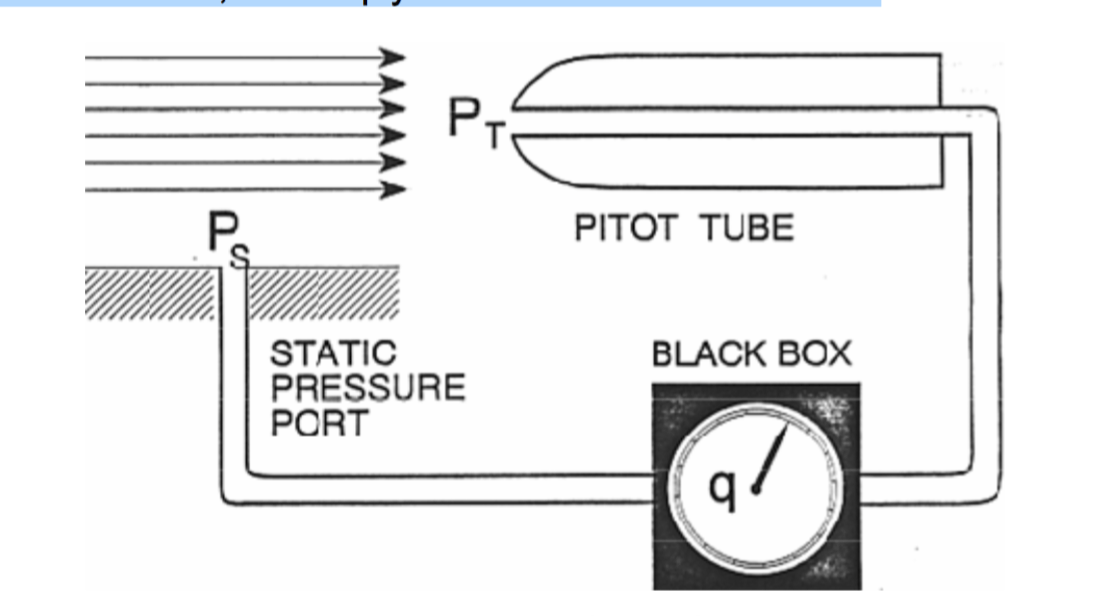
Airspeed Measurement
admin@readysettakeoff.com
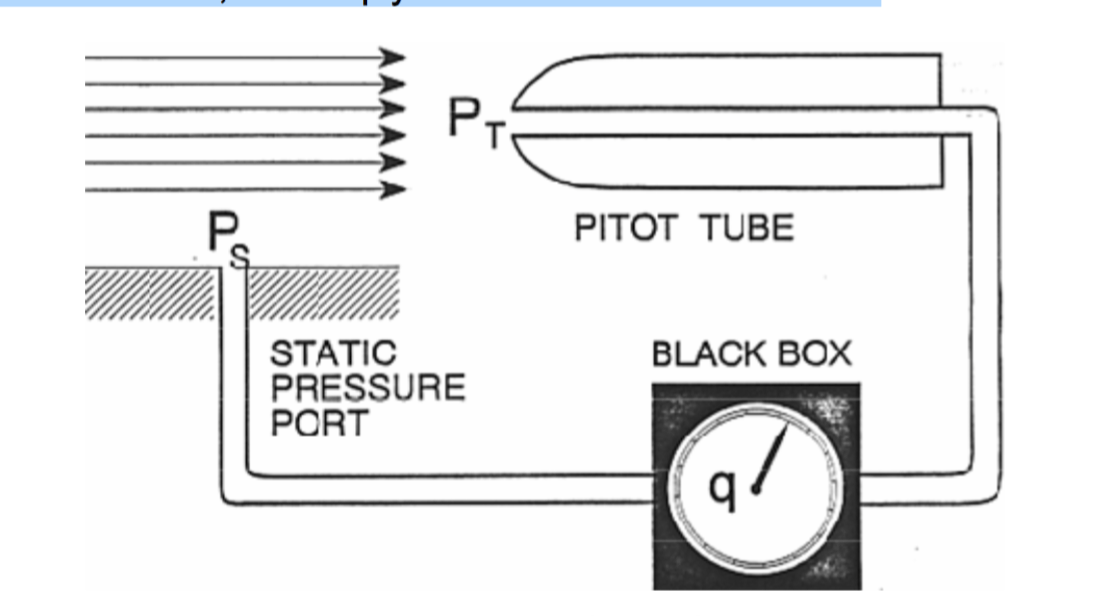
Related Articles
-
Factors Affecting lift
Jet Brain, , Aerodynamics, 0
You are unauthorized to view this page. Please login your credentials Username Password Remember Me Forgot Password
-
Turn Performance
Jet Brain, , Aerodynamics, 0
You are unauthorized to view this page. Please login your credentials
-
Stall Pattern and Wing Design
Jet Brain, , Aerodynamics, 0
You are unauthorized to view this page. Please login your credentials
-
Camber Change
Jet Brain, , Aerodynamics, 0
You are unauthorized to view this page. Please login your credentials
-
Wing Dihedral
Jet Brain, , Aerodynamics, 0
You are unauthorized to view this page. Please login your credentials
-
Takeoff Performance
Jet Brain, , Aerodynamics, 0
You are unauthorized to view this page. Please login your credentials
-
Stalls
Jet Brain, , Aerodynamics, 0
You are unauthorized to view this page. Please login your credentials
-
Basic Aircraft Review
Jet Brain, , Aerodynamics, 0
You are unauthorized to view this page. Please login your credentials