In the preceding discussion of lift, an assumption was made
that air was an ideal fluid, with no viscosity or friction effects.
In actually, when air flows across any surface, friction develops. The air immediately next to the surface slows to near zero velocity as it gives up kinetic energy to friction. As a viscous fluid resists flow or shearing, the adjacent layer of air is also slowed. Succeeding streamlines are slowed less, until eventually some outer streamline reaches the free airstream velocity.
The boundary layer is that layer of airflow over a surface that demonstrates local airflow retardation due to viscosity. It is usually no more than 1mm thick (the thickness of a playing card) at the leading edge of an airfoil, and grows in thickness as it moves aft over the surface. The boundary layer has two types of airflow.
In laminar flow, the air moves smoothly along in streamlines. A laminar boundary layer produces very little friction, but is easily separated from the surface.
In turbulent flow, the streamlines break up and the flow is disorganized and irregular. A tur- bulent boundary layer produces higher friction drag than a laminar boundary layer, but adheres better to the upper surface of the airfoil, delaying boundary layer separation.
Any object that moves through the air will develop a boundary layer that varies in thickness according to the type of surface. The type of flow in the boundary layer depends on its location on the surface. The boundary layer will be laminar only near the leading edge of the airfoil. As the air flows aft, the laminar layer becomes turbulent. The turbulent layer will continue to increase in thickness as it flows aft.
As air flows aft from the leading edge of the airfoil, it moves from a high-pressure area towards the low-pressure area at the point of maximum thickness. This favorable pressure gradient assists the boundary layer in adhering to the surface by maintaining its high kinetic energy. As the air flows aft from the point of maximum thickness (lower static pressure) toward the trailing edge (higher static pressure), it encounters an adverse pressure gradient which impedes the flow of the boundary layer.
The adverse pressure gradient is strongest at high lift conditions, and at high angles of attack in particular. If the boundary layer does not have sufficient kinetic energy to overcome the adverse pressure gradient, the lower levels of the boundary layer will stagnate. The boundary layer will then separate from the surface, and airflow along the surface aft of the separation point will be reversed. Aft of the separation point, the low static pressure that produced lift is replaced by a turbulent wake.
If the separation point moves forward enough close to the leading edge, the net suction on the top of the airfoil will decrease and a decrease in CL will occur, resulting in a stall. The angle of attack beyond which CL begins to decrease is CLmax AOA. Even at low angles of attack there will be a small adverse pressure gradient behind the point of maximum thickness, but it is insignificant compared to the kinetic energy in the boundary layer until CLmax AOA is approached.
The point of separation remains essentially stationary near the trailing edge of the wing, until AOA approaches CLmax AOA. The separation point then progresses forward as AOA increases, eventually causing the airfoil to stall. At high angles of attack the airfoil is similar to a flat plate being forced through the air; the airflow simply cannot conform to the sharp turn. Note that the point where stall occurs is dependent upon AOA and not velocity.
A stall is a condition of flight in which an increase
in AOA results in a decrease in CL. The highest value of CL is referred to as CLmax, and any increase in AOA beyond CLmax AOA produces a decrease in CL. Therefore, CLmax AOA is known as the stalling angle of attack or critical angle of attack, and the region beyond CLmax AOA is the stall region. Regardless of the flight conditions or airspeed, the wing will always stall at the same AOA, CLmax. The only cause of a stall is excessive AOA. Stalls result in decreased lift, increased drag, and an altitude loss. They are particularly dangerous at low altitude or when allowed to develop into a spin. The only action necessary for stall recovery is to decrease AOA below CLmax AOA.
STALL SPEED
As angle of attack increases, up to CLmax AOA, true airspeed decreases in level flight. Since CL decreases beyond CLmax AOA, true airspeed cannot be decreased any further. Therefore the minimum airspeed required for level flight occurs at CLmax AOA. Stall speed (VS) is the minimum true airspeed required to maintain level flight at CLmax AOA. Although the stall speed may vary, the stalling AOA remains constant for a given airfoil. Since lift and weight are equal in equilibrium flight, weight (W) can be substituted for lift (L) in the lift equation. By solving for velocity (V), we derive a basic equation for stall speed.
By substituting the stall speed equation into the true airspeed equation and solving for
indicated airspeed, we derive the equation for the indicated stall speed (IASS). Weight, altitude, power, maneuvering, and configuration greatly affect an airplane’s stall speed. Maneuvering will increase stall speed, but will not be discussed until the lesson that deals with turning flight.
As airplane weight decreases stall speed decreases because the amount of lift required to maintain level flight decreases. When an airplane burns fuel or drops ordnance, stall speeds decrease. Carrier pilots often dump fuel before shipboard landings in order to reduce stall speed and approach speed.
A comparison of two identical airplanes at different altitudes illustrates the effect of altitude on stall speed. The airplane at a higher altitude encounters fewer air molecules. In order to create sufficient dynamic pressure to produce the required lift, it must fly at a higher velocity (TAS). Therefore, an increase in altitude will increase stall speed. Since ρ0 is constant, indicated stall speed will not change as altitude changes.
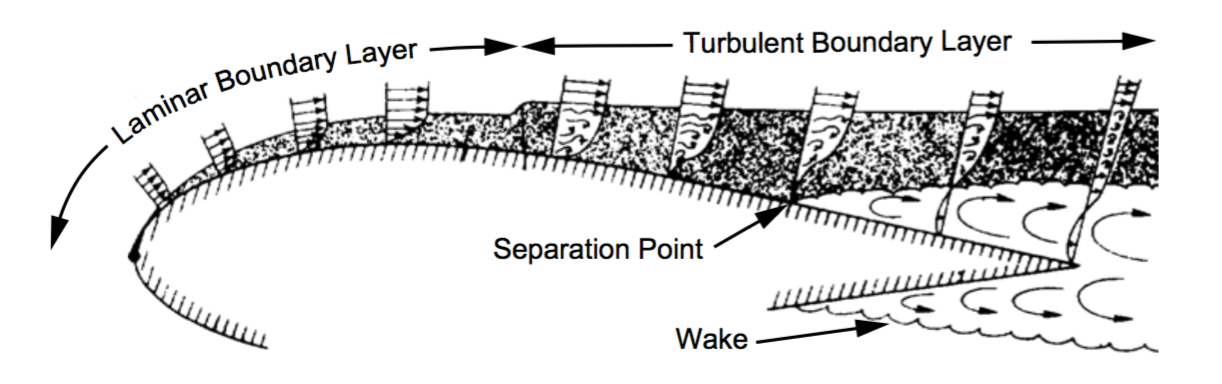